The following is the established format for referencing this article:
Blake, J. G. 2024. Vocal activity of lowland forest birds in eastern Ecuador varies by foraging strata, guild, and species during the first hours of the morning. Journal of Field Ornithology 95(3):12.ABSTRACT
Patterns of vocal activity vary among tropical bird species, with some tending to sing before or close to dawn (“dawn chorus”) whereas others are more likely to vocalize later in the morning. Timing of vocal activity can, therefore, affect the results of bird counts which often rely heavily on vocalizations for species identification. Passive acoustic monitoring (PAM), which uses autonomous recording units (ARUs) to record vocalizations at a set schedule, allows birds to be sampled at multiple points simultaneously and can be set to record over extended time periods at single points. Thus, monitors provide an effective way to document vocal activity patterns during the morning when birds are typically most active. I used ARUs to record vocal activity of birds at a lowland forest site in eastern Ecuador during 2013-2017 on two 100-ha study plots. Monitors were set to record for 10-min periods followed by a 5-min break from 0545 to 0810. Species were identified by listening to the recordings, with presence of species noted during each 10-min period. Activity (number of species occurrences per period) was examined by strata (understory, canopy), guild, and by individual species. Overall patterns of activity (all species combined) increased rapidly from before dawn to about 0630 and then gradually decreased. The pattern was the same on both plots and consistent across years on each plot. Activity patterns differed among strata, guilds, and individual species. Understory birds peaked in activity before canopy birds and then declined to a point where there was less vocal activity than among canopy birds. Terrestrial granivores, omnivores, and frugivores all showed an early morning peak followed by a rapid decrease in contrast to arboreal species that increased in activity throughout the morning. Terrestrial insectivores did not differ from bark insectivores in their patterns of activity even though bark insectivores forage at higher strata. Substantial variation among species within different guilds also was apparent and illustrates that patterns of activity can vary even among species that forage in similar ways. Passive acoustic monitoring is a useful method for sampling bird activity because multiple monitors can be active at the same time across multiple points.
RESUMEN
Los patrones de actividad vocal varían entre las especies de aves tropicales, con algunas tendiendo a cantar antes o cerca del amanecer (“coro del amanecer”) mientras que otras son más propensas a vocalizar más tarde en la mañana. El momento de la actividad vocal puede, por lo tanto, afectar los resultados de los conteos de aves, que a menudo dependen en gran medida de las vocalizaciones para la identificación de las especies. Los monitoreos acústicos pasivos (MAP), los que utilizan unidades de grabación autónoma (UGAs) para grabar las vocalizaciones en un esquema de horarios establecido, permiten muestrear a las aves en diferentes puntos simultáneamente y configurar los equipos para grabar durante períodos de tiempo prolongados en cada punto. Así, este monitoreo proporciona una forma efectiva para documentar los patrones de actividad vocal durante la mañana, cuando las aves están típicamente más activas. Utilicé UGAs para grabar la actividad de las aves en un sitio de bosques de tierras bajas en el este de Ecuador durante 2013-2017 en dos parcelas de estudio de 100 ha. Las UGAs fueron configuradas para grabar durante periodos de 10 min seguidos de una pausa de 5 min, entre las 0545 y las 0810. Las especies fueron identificadas mediante la escucha de las grabaciones, registrando la presencia de las especies en cada período de 10 min. La actividad (número de especies por período) fue examinada por estratos (sotobosque, dosel), gremios, y especies. Los patrones de actividad general (todas las especies combinadas) aumentaron rápidamente desde antes del amanecer hasta cerca de las 0630 y luego decrecieron gradualmente. El patrón fue el mismo en las dos parcelas y consistente a lo largo de los años en cada parcela. Los patrones de actividad difirieron entre estratos, gremios y especies. Las aves del sotobosque alcanzaron un punto máximo de actividad antes que las aves de dosel y luego declinaron hasta un punto donde presentaron menos actividad vocal que las aves de dosel. Los granívoros terrestres, omnívoros y frugívoros mostraron un punto máximo de actividad temprano en la mañana seguido por un rápido decrecimiento en contraste con las especies arbóreas que incrementaron su actividad a lo largo de la mañana. Aunque los insectívoros de corteza forrajean en un estrato más alto, no difirieron de los insectívoros terrestres en sus patrones de actividad. También se encontró una considerable variación entre especies dentro de los diferentes gremios, lo que muestra que los patrones de actividad pueden variar aún entre especies que forrajean de forma similar. El monitoreo acústico pasivo es un método adecuado para muestrear la actividad de las aves puesto que múltiples unidades de grabación pueden permanecer activas al mismo tiempo en diferentes puntos.
INTRODUCTION
Passive acoustic monitoring (PAM), which uses autonomous recording units (ARUs), can be an effective method for sampling birds and other organisms (Aide et al. 2017, Sugai et al. 2019, Blake 2021, Ross et al. 2023). Passive acoustic monitoring has been used to assess bird species richness and abundance (Celis-Murillo et al. 2009, Ribeiro et al. 2017), to conduct rapid inventories of bird species (Stevens et al. 2019), to estimate density (Sebastián-González et al. 2018; refer to review in Pérez-Granados and Traba 2021), and to determine effective sampling schemes (de Araújo et al. 2021, Metcalf et al. 2022). Use of ARUs has been suggested as an alternative to point counts for avian monitoring (Alquezar and Machado 2015, Leach et al. 2016, Darras et al. 2018a) and they have proven useful for assessing effects of habitat disturbance, such as gold mining and habitat fragmentation (Alvarez-Berríos et al. 2016, de Camargo et al. 2019). Acoustic indices, derived from ARU recordings, have also been suggested as a proxy for biodiversity (Jorge et al. 2018, Alocer et al. 2022). Their effectiveness for biodiversity monitoring has, however, been questioned (Bicudo et al. 2023). Changes in bird abundance and distribution are often evaluated based on differences in numbers of calls (vocal activity rate, detection rate; Hutschenreiter et al. 2024). These new indices may prove valuable for assessing relative abundance.
ARUs have the benefit that multiple locations can be sampled simultaneously, unlike point counts which typically are restricted to sampling a single point at a time. By spreading monitors over a wide area, temporal and spatial variation in vocal activity can be assessed. Tropical bird species often differ in temporal patterns of vocalization (Parker 1991, Blake 1992, Antunes 2008, Hart et al. 2015, Oliveira et al. 2023) with the dawn chorus typically a time of peak activity for many species. Some species only sing just before dawn (e.g., some woodcreepers, tinamous, ovenbirds) whereas others typically sing later in the morning (e.g., many parrots, toucans; Blake 1992). Given that birds vary in fine-scale spatial distribution patterns [e.g., in response to small-scale variation in habitat (Menger et al. 2017)], the number and identity of species vocalizing at a given point will vary both with time and space. Birds also may vary their use of space and time to minimize acoustic interference with other birds or insects (Luther 2009; Tobias et al. 2014). Most bird species are not uniformly distributed across habitats and microhabitats (e.g., Menger et al. 2017, dos Anjos et al. 2022) so sampling multiple points simultaneously, as is possible with ARUs, may provide a better understanding of how vocal activity varies over time and space. Such knowledge is particularly important for species that are patchily distributed and/or that limit their vocal activity to specific times in the morning. Yet, there have been few studies that have used PAM to examine temporal variation in vocal activity (de Araújo et al. 2023, 2024, Metcalf et al. 2022).
Here, I use data from a study conducted in lowland forest of eastern Ecuador that used ARUs to sample birds on two 100-ha study plots (Blake 2021). Monitors acted as point counts, sampling birds for ten 10-min periods starting at 0545, when nocturnal species were still vocalizing and diurnal birds were starting to sing. My major objectives were to examine how and if vocal activity varied over time (over two hours in the morning, across years) and space (between plots) for birds that use different foraging strata (i.e., canopy vs. understory), for foraging guilds (e.g., insectivore, frugivore), and for individual species. To my knowledge, this is the first study to have examined vocal activity patterns across multiple points, plots, and years. Thus, this study is unique in the temporal and spatial coverage of activity patterns of multiple species in a diverse lowland Neotropical forest.
METHODS
Study site
Research was conducted at Tiputini Biodiversity Station (TBS), Orellana Province, Ecuador (ca 0°37′ S, 76°10′ W, 190–270 meters above sea level). TBS is located on the north bank of the Tiputini River, bordering Yasuní National Park and within Yasuní Biosphere Reserve, one of the most diverse regions of the world (Bass et al. 2010). The station and nearby areas are dominated by terra firme forest (Bredin et al. 2020); várzea forest, palm swamps, and various successional habitats also are present. Mean annual precipitation at Yasuní Research Station, approximately 30 km WSW of TBS, is about 3100 mm (Blake et al. 2011).
Two ca 100-ha plots (ca 1 km x 1 km each) were established in terra firme forest during 2001. Both plots are gridded (100-m east-west x 200-m north-south grid lines) and marked with 1.5-m PVC tubes at 50-m intervals. The Harpia plot ranges from ~201 to 233 m elevation and is characterized by more dissected upland forest. The Puma plot is flatter overall although elevation range is similar, from ~209 to 235 m. Flat areas on Puma may have pools of standing water after prolonged, heavy rains. Dominant vegetation on both plots is tall, evergreen forest. Treefalls are a common occurrence and cause local, small-scale variation in habitats that may influence distribution patterns of some species. Overall patterns of diversity and abundance of birds are similar on the two plots (Blake 2007, 2021, Blake and Loiselle 2009, 2015) but there are differences in the distribution and abundance patterns of individual species. Those differences might be expected to influence vocal activity patterns.
Bird sampling
Birds were sampled during January–March, 2013–2017, with acoustic monitors (Song Meter SM2+, Wildlife Acoustics, Inc., Maynard, MA, USA) equipped with two SMX-II omnidirectional microphones. Monitors were attached to trees ~1.5 m above ground along transects on each plot, with monitors 200–225 m apart. Five monitors were deployed simultaneously on each plot (i.e., 10 ARUs/day) on transects located 200 m apart (e.g., on east-west transects). Monitors were left in place until two mornings without rain had elapsed and were then moved 200 m east (or west, depending on the plot) to alternate transects until 25 separate points were sampled on each plot (Fig. 1). Monitors were set to record for 10 min followed by a 5-min break, starting at 0545 hr and ending at 0810 hr, for a total of 10 recording sessions in a morning. I set monitors to record at a sampling rate of 16 kHz, providing a detection window up to 8 kHz, which encompassed the great majority of bird vocalizations, particularly those in the understory and louder canopy species (Dooling 2004, Weir et al. 2012). Aide et al. (2017), for example, found that most bird vocalizations were less than 8 kHz. Thus, although monitors likely missed some species, particularly canopy species with high frequency or quiet songs, they sampled most birds whose vocalizations were detectable by recorders placed close to ground level.
Recordings were manually reviewed to identify species; identifications were based on my knowledge of bird songs and calls and by comparisons to published songs and calls from birds in Ecuador. I also used Song Scope 4.1.5 (Wildlife Acoustics, Inc., Maynard, MA, USA) to visualize spectrograms of the different calls and songs, which aided identifications. No attempt was made to determine numbers of individuals recorded per species in a given 10-min period nor to estimate distance; thus, all analyses are based on numbers and identities of species per recording period.
Analyses
Numbers of species identified from recordings were summarized by point and time for one day of sampling per point per year. Time constraints precluded using both days of recordings. Further, only 14 points were sampled on Puma during 2013 because of time constraints and data from 2016 on Puma were not included as most recorders failed to work properly. When summarizing data from one period (i.e., 10-min interval), I only counted a given species, including unidentified species, once no matter how many times the species vocalized during the count period. Vocal activity was assessed based on the number of 10-min periods during which a species was identified. With 25 points and ten 10-min periods, a given species could be counted as present a maximum of 250 times per plot per year. The actual number of records was always less as no species vocalized at all points and during all 10-min periods. Species were classified by foraging strata (canopy, including subcanopy; understory, including ground) following Karr et al. (1990) and by guild (arboreal frugivore; terrestrial frugivore; arboreal granivore; terrestrial granivore; arboreal omnivore; bark insectivore, including trunk and superficial surface; terrestrial insectivore, including gleaning and sallying; arboreal gleaning insectivore; arboreal sallying insectivore) following Terborgh et al. (1990) to examine the effects of strata and resource use on vocal activity. A previous study (Blake 1992) demonstrated distinct differences in vocal activity of canopy and understory species.
Correlation coefficients were used to compare patterns of activity between plots, between strata, and among guilds between plots. Analyses were conducted with Statistix 10.0 (Analytical Software 2013). Species level comparisons were based on data combined across plots.
RESULTS
I accumulated 16,631 records of both identified and unknown species summed across points on Harpia across all years (2013 - 3276; 2014 - 3570; 2015 - 3387; 2016 - 3299; 2017 - 2099) and 11,465 on Puma (2013 - 2098; 2014 - 3183; 2015 - 3334; 2017 - 2850). Refer to Appendix 1 for a complete list of species by plot.
Number of records, expressed as percentage of total records, increased rapidly from 0545 to about 0630 before gradually decreasing on both plots (Fig. 2). With all years combined, the pattern was highly correlated between plots (r = 0.99, p < 0.001). Similar patterns were observed every year, with correlations between years on a given plot and between plots for years separately > 0.95 in almost all cases; correlation between 2013 and 2014 on Harpia was 0.87.
Given the lack of variation among years, I combined data across years to examine patterns by strata and guild. Unidentified vocalizations accounted for from 4.5 to 8.5% of all records and were not included in these analyses. Vocal activity of understory birds increased rapidly from 0545 to about 0630 before decreasing (Fig. 3); the pattern was the same on both plots (r = 0.96, p < 0.001). Vocal activity of canopy birds was lower than that of understory birds until about 0645 but did not show the same pattern of decreased activity later in the morning. Again, patterns were the same on both plots (r = 0.98, p < 0.001). Vocal activity of understory and canopy birds were less correlated with each other, particularly on Puma (canopy vs. understory: Harpia, r = 0.65, p < 0.05; Puma, r = 0.32, p = 0.38).
Vocal activity patterns of guilds were, with one exception, highly correlated (r > 0.90, p < 0.001) between plots (Fig. 4). The lone exception was for terrestrial frugivores which showed a somewhat different pattern between plots (r = 0.49, p = 0.149). That difference partially reflected activity patterns of Mitu salvini, which was more commonly recorded on Puma and which primarily sang just before dawn, and Geotrygon montana, which was more common on Harpia and which sang more frequently later in the morning (Fig. 5).
Patterns of activity were similar for some guilds but differed among others. For example, percentage of records for arboreal frugivores was lower than for terrestrial frugivores until about 0645; arboreal frugivore activity continued to increase throughout the sample period whereas terrestrial frugivores decreased (Fig. 4a). Terrestrial granivores showed a rapid increase in activity until 0615 but then showed a rapid decrease until 0800 (Fig. 4b). In contrast, arboreal granivores and arboreal omnivores increased in activity throughout the morning. Bark insectivores and terrestrial insectivores both increased rapidly until 0630 before declining gradually (Fig. 4c). Finally, arboreal sallying insectivores and arboreal gleaning insectivores showed similar overall patterns, with gleaning insectivores showing less activity early on and slightly greater activity later in the morning (Fig. 4d).
Number of records per species was highly correlated between plots (r = 0.84, p < 0.000, 217 species) and results from the two plots were combined to examine overall patterns. Most species were represented by relatively few records (Table 1). Only 12 species were represented by 500 or more records whereas 114 species were represented by < 50 records. There were 62 species with 10 or fewer records (Table 1), accounting for 28.6% of species but only 1.0% of records.
Activity patterns for individual species were examined for species with at least 150 records. Although some species within guilds showed similar patterns of activity (Figs. 5, 6), others did not. For example, large canopy frugivores (Fig. 5a) had very similar patterns, generally increasing throughout the morning. In contrast, among arboreal frugivores in the subcanopy or understory, Lepidothrix coronata showed lower activity early in the morning compared to other species (Fig. 5b) but higher activity later (from 0730 on). Arboreal granivores (Fig. 5c) showed more similar patterns to each other than did terrestrial granivores (Fig. 5d). Crypturellus variegatus, for example, was more active than other terrestrial granivores during the first two count periods before declining to a low level at the end of the morning (Fig. 5d). In contrast, Tinamus major increased activity rapidly during the first three periods but then rapidly declined. Some arboreal omnivores showed very different patterns (Fig. 5e) whereas others showed more consistent patterns of activity (Fig. 5f). Mitu salvini, a terrestrial frugivore, provides an illustration of species whose activity is highest just before dawn but which falls rapidly afterwards; Geotrygon montana, in contrast, gradually increased in activity and then gradually decreased (Fig. 5g).
Insectivores also showed a variety of patterns within guilds (Fig. 6). Among bark insectivores, two woodcreepers showed higher activity levels earlier in the morning than did two woodpeckers (Fig. 6a). Three terrestrial insectivores, in contrast, showed similar patterns of activity during the morning (Fig. 6b). Arboreal sallying insectivores showed a variety of different patterns; Thamnomanes ardesiacus and T. caesius are both important members of mixed-species flocks but showed distinctly different patterns of vocal activity (Fig. 6c). Some arboreal gleaning insectivores had similar patterns of activity (Fig. 6d, f) whereas others did not (Fig. 6e, g). Pygiptila stellaris (Fig. 6e), for example, showed a much higher peak of activity early in the morning compared to others. Three Myrmotherula antwrens (Fig. 6g) showed different patterns although all are common in mixed-species flocks.
DISCUSSION
Patterns of overall vocal activity of birds during early morning (first two hours) on two 100-ha study plots in lowland eastern Ecuador were very similar both between plots and across years. There was a rapid increase in total activity from just before dawn until approximately 1 hour after dawn after which activity remained relatively constant. Yet, that consistency in overall numbers of detections obscured substantial variation among different strata, guilds, and species. Understory birds, for example, peaked in activity before canopy birds and declined to a point where there was less activity than among canopy birds. This follows a similar pattern found in an earlier study in Costa Rica (Blake 1992). Similarly, terrestrial granivores, omnivores, and frugivores all showed an early morning peak followed by a rapid decrease in contrast to arboreal species that increased in activity throughout the morning. Terrestrial insectivores, on the other hand, did not differ from bark insectivores in their patterns of activity even though bark insectivores forage at higher strata. Substantial variation among species within different guilds also was apparent and illustrates that patterns of activity can vary even among species that forage in similar ways.
Total activity on both plots remained fairly constant with a slight decrease after about 0630. Similarly, number of species recorded during different time periods also remained fairly constant after ~0630, based on the same data (Blake 2021). Yet, species accumulation curves based on these same data continued to increase throughout the morning (Blake 2021) indicating turnover in species composition during the different times of the morning. This turnover reflects the different patterns of activity among species, with some increasing in activity earlier in the morning and some later. A similar pattern of turnover in species composition was seen by Oliveira et al. (2023), who found a peak in richness early in the morning with few additional species counted after the first two hours.
Temporal variation in detectability is an important consideration when sampling birds because species differ in timing of activity (Skutch 1954, 1960, Parker 1991, Blake 1992, Metcalf et al. 2022, de Araújo et al. 2024, Hopping et al. 2024). Some species tend to sing mostly just before or near dawn (e.g., Baryphthengus martii, Bucco capensis, Dendrexetastes rufigula, Micrastur spp., Mitu salvini); others primarily within the first hour or so after dawn (e.g., Thamnomanes ardesiacus, Thamnophilus murinus, Tinamus major, Turdus albicollis); and others with greater activity later in the morning (e.g., Ara macao and other psittacids, Pachysylvia hypoxantha, Lepidothrix coronata, Querula purpurata, Ramphastos spp.). Thus, timing of counts may depend on whether a study is focused on specific species or the entire community. De Araújo et al. (2021) found that most birds called between 0500 and 0700 at a site in Atlantic rainforest of Brazil and concluded that was the most effective sampling period for a community study; that study was, however, based on recordings at a single site over five days in one year. Hopping et al. (2024) demonstrated significant temporal variation in vocal activity of birds both within the dawn hour and across days. As demonstrated with this study, some species are more active later in the morning and may not be detected if count periods are too short. Given the turnover in species composition through the morning, longer count periods may be needed to ensure a more complete enumeration of species. Continuing counts for at least two hours after sunrise, as in this study, may allow additional species to be detected. Oliviera et al. (2023) found that the first 1 hr 45 min of the morning was the best time for sampling most species but that longer periods could be needed to increase the chances of recording locally rare species. Similarly, because community composition can vary substantially across years (e.g., Blake and Loiselle 2015, 2024, Stouffer et al. 2020, Pollock et al. 2022), sampling vocalizations across multiple years may also provide a better description of variation in temporal vocalization patterns. In addition, because some species may be locally rare (Terborgh et al. 1990, Oliviera et al. 2023) and recorded at relatively few points (Blake 2021), many points may need to be sampled to achieve a full enumeration of species present in a study area.
Patterns of activity may reflect a variety of different influences that may affect species in different ways. For example, differences in activity patterns among guilds suggest that foraging behavior may have an influence on patterns of activity, perhaps reflecting availability of prey items. Early morning hours may also be better for sound transmission in dense tropical forests because background noise may be lower and the broadcast area greater than later in the morning (Henwood and Fabrick 1979), which might help explain why vocal activity of many species is higher early in the morning, particularly in the dense understory. Further, birds are known to adjust timing and rate of vocalizations to avoid overlap/interference with other species (Luther 2009). For example, timing of activity may reflect the need to avoid overlap with insect vocalizations which may interfere with transmission (or reception) of bird vocalizations. Hart et al. (2015) found that birds avoid overlap with vocalizations of a cicada (Zammara smaragdina). In that study, birds vocalized with little interference during the first 2–3 hr after dawn but number of vocalizations and number of species vocalizing dropped significantly after cicadas started making noise after about 0840 in the morning.
Bird species in tropical forests often are rare and/or are spatially restricted by habitat/microhabitat conditions (Terborgh et al. 1990, Robinson et al. 2000, Blake and Loiselle 2009, Bueno et al. 2012, Pomara et al. 2012, Menger et al. 2017). Point counts often may not encounter such spatially restricted species given that counts typically do not simultaneously cover multiple points. ARUs, on the other hand, can be deployed across multiple points at the same time, recording species vocalizations over a greater area and increasing the potential to encounter species that occur at few points. In this study, almost 30% of species were recorded fewer than 10 times; many were recorded at only one point (Blake 2021). Spacing of ARUs will affect probability of detection of different species, given that detection ranges can vary among species, among habitats, and with differences in weather conditions (e.g., Darras et al. 2016, Winiarska et al. 2024). Thus, knowledge of detection ranges of different species may be useful for determining the appropriate spacing pattern for ARUs, depending on the study objectives (Darras et al. 2018b). As with point counts, if ARUs are too close together there is the possibility that some species may be detected simultaneously at more than one recorder. In the current study, ARUs were at least 200 m apart, a typical spacing for point counts and one that is likely to preclude double-counting most species. PAM also benefits from the ability to simultaneously sample multiple points for several hours in the morning and, as a consequence, may be more likely to detect species at a given point, particularly species that only sing for brief periods (e.g., Dendrexetastes rufigula, Bucco capensis).
CONCLUSIONS
Tropical lowland forests are among the most diverse regions for birds, whose vocal activity can vary tremendously. Thus, when designing studies to sample birds, knowledge of the patterns of vocal activity can be important, particularly given that most identifications of birds in tropical forest surveys are based on auditory contacts rather than visual (personal observation). PAM provides a mechanism for assessing variation in activity patterns of multiple species and can do so over wider areas and longer periods than are typical for assessments based on point counts. Overall patterns of activity in this study were very consistent across years and between plots separated by about 1.5 km, suggesting that vocal activity is predictable at some scales. For example, samples early in the morning, typically starting before dawn, are likely to record the most species (Blake 1992, Antunes 2008, de Araújo et al. 2021) although they may miss some species that start to sing later in the morning. Results of this study clearly demonstrated that vocal activity varies among groups that differ in foraging behavior (strata used, diet). Yet, individual species within such groups often display different patterns of vocal activity, making generalizations about species within a guild or other group problematic. Causes of such variation in behavior may be related to factors that influence sound transmission and reception, such as habitat structure, sounds from insects or other organisms that mask bird sounds (Hart et al. 2015) or interference from other species in production and reception of sounds (Luther 2008, 2009). Additional studies would be needed to parse out the influence of such factors on vocal activity of birds.
RESPONSES TO THIS ARTICLE
Responses to this article are invited. If accepted for publication, your response will be hyperlinked to the article. To submit a response, follow this link. To read responses already accepted, follow this link.
ACKNOWLEDGMENTS
I am grateful to the individuals who helped establish the 100-ha study plots. I also thank the staff of the Tiputini Biodiversity Station, especially D. Mosquera, G. Vinueza, C. de Romo, D. Romo, K. Swing, and all others who have made visits to the site so rewarding. B. A. Loiselle made useful comments on an earlier version of this paper as did three anonymous reviewers. Funding for this study was provided by the University of Florida and personal sources. Approval for this research was obtained from Institutional Animal Care and Use Committee, University of Florida Non-Regulatory Animal Research Committee (#201710065). Work at Tiputini Biodiversity Station was conducted in accordance with research permit number 025-2019-IC-PNY-DPAO (and earlier ones), Ministerio del Ambiente, Puerto Francisco de Orellana, Ecuador.
DATA AVAILABILITY
Data will be made available upon reasonable request.
LITERATURE CITED
Aide, T. M., A. Hernández-Serna, M. Campos-Cerqueira, O. Acevedo-Cherry, and J. L. Deichmann. 2017. Species richness (of insects) drives the use of acoustic space in the tropics. Remote Sensing 9(11):1096 https://doi.org/10.3390/rs9111096
Alocer, I., H. Lima, L. S. M. Sugai, and D. Llusia. 2022. Acoustic indices as proxies for biodiversity: a meta-analysis. Biological Review 97(6):2209-2236. https://doi.org/10.1111/brv.12890
Alquezar, R. D., and R. B. Machado. 2015. Comparisons between autonomous acoustic recordings and avian point counts in open woodland savanna. Wilson Journal of Ornithology 127(4):712-723. https://doi.org/10.1676/14-104.1
Alvarez-Berríos, N., M. Campos-Cerqueira, A. Hernández-Serna, C. J. A. Delgado, F. Román-Dañobeytia, and T. M. Aide. 2016. Impacts of small-scale gold mining on birds and anurans near the Tambopata Natural Reserve, Peru, assessed using passive acoustic monitoring. Tropical Conservation Science 9(2):832-851. https://doi.org/10.1177/194008291600900216
Analytical Software. 2013. Statistix, Version 10.0. Analytical Software, Tallahassee, Florida, USA.
Antunes, A. Z. 2008. Diurnal and seasonal variability in bird counts in a forest fragment in southeastern Brazil. Revista Brasileira de Zoologia 25(2):228-237. https://doi.org/10.1590/S0101-81752008000200011
Bass, M. S., M. Finer, C. N. Jenkins, H. Kreft, D. F. Cisneros-Heredia, S. F. McCracken, N. C. A. Pitman, P. H. English, K. Swing, G. Villa, A. Di Fiore, C. C. Voigt, and T. H. Kunz. 2010. Global conservation significance of Ecuador’s Yasuní National Park. PLoS ONE 5:e8767. https://doi.org/10.1371/journal.pone.0008767
Bicudo, T., D. Llusia, M. Anciãs, and D. Gil. 2023. Poor performance of acoustic indices as proxies for bird diversity in a fragmented Amazonian landscape. Ecological Informatics 77:102241 https://doi.org/10.1016/j.ecoinf.2023.102241
Blake, J. G. 1992. Temporal variation in point counts of birds in a lowland wet forest in Costa Rica. Condor 94(1):265-275. https://doi.org/10.2307/1368816
Blake, J. G. 2007. Neotropical forest bird communities: a comparison of species richness and composition at local and regional scales. Condor 109(2):237-255. https://doi.org/10.1093/condor/109.2.237
Blake, J. G. 2021. Acoustic monitors and direct observations provide similar but distinct perspectives on bird assemblages in a lowland forest of eastern Ecuador. PeerJ 9:e10565 https://doi.org/10.7717/peerj.10565
Blake, J. G., and B. A. Loiselle. 2009. Species composition of Neotropical understory bird communities: local versus regional perspectives based on capture data. Biotropica 41(1):85-94. https://doi.org/10.1111/j.1744-7429.2008.00445.x
Blake, J. G., and B. A. Loiselle. 2015. Enigmatic declines in bird numbers in lowland forest of eastern Ecuador may be a consequence of climate change. PeerJ 3:e1177. https://doi.org/10.7717/peerj.1177
Blake, J. G., and B. A. Loiselle. 2024. Sharp declines in observation and capture rates of Amazon birds in absence of human disturbance. Global Ecology and Conservation 51:e02902. https://doi.org/10.1016/j.gecco.2024.e02902
Blake, J. G., D. Mosquera, J. Guerra, B. A. Loiselle, D. Romo, and K. Swing. 2011. Mineral licks as diversity hotspots in lowland forest of eastern Ecuador. Diversity 3(2):217-234. https://doi.org/10.3390/d3020217
Bredin, Y. K., J. E. Hawes, C. A. Peres, and T. Haugaasen. 2020. Structure and composition of terra firme and seasonally flooded várzea forests in the western Brazilian Amazon. Forests 11(12):1361 https://doi.org/10.3390/f11121361
Bueno, A. S., R. S. Bruno, T. P. Pimentel, T. M. Sanaiotti, and W. E. Magnusson. 2012. The width of riparian habitats for understory birds in an Amazonian forest. Ecological Applications 22(2):722-734. https://doi.org/10.1890/11-0789.1
Celis-Murillo, A., J. L. Deppe, and M. F. Allen. 2009. Using soundscape recordings to estimate bird species abundance, richness, and composition. Journal of Field Ornithology 80(1):64-78. https://doi.org/10.1111/j.1557-9263.2009.00206.x
Darras, K., Pütz, Fahrurrozi, K. Rembold, and T. Tscharntke. 2016. Measuring sound detection spaces for acoustic animal sampling and monitoring. Biological Conservation 201:29-37. https://doi.org/10.1016/j.biocon.2016.06.021
Darras, K., P. Batáry, B. Furnas, A. Celis-Murillo, S. L. Van Wilgenberg, Y. A. Mulyani, and T. Tscharntke. 2018a. Comparing the sampling performance of sound recorders versus point counts in bird surveys: a meta-analysis. Journal of Applied Ecology 55(6):2575-2586. https://doi.org/10.1111/1365-2664.13229
Darras, K., B. Furnas, I. Fitriawan, Y. Mulyani, and T. Tscharntke. 2018b. Estimating bird detection distances in sound recordings for standardizing detection ranges and distance sampling. Methods in Ecology and Evolution 9(9):1928-1938. https://doi.org/10.1111/2041-210X.13031
De Araújo, C. B., M. Jardim, N. dos S. F. Saturnino, G. M. Rosa, M. R. Lima, and L. dos Anjos. 2021. The optimal listening period for an effective assessment of bird richness and composition: a case study of Neotropical forest. Journal of Ornithology 162:303-306. https://doi.org/10.1007/s10336-020-01812-6
De Araújo, C. B., M. R. Lima, P. Albuquerque, R. D. Alquezar, M. Barreiros, M. Jardim, E. Gangenova, R. B. Machado, B. T. Phalan, A. L. Roos, G. L. M. Rosa, N. Saturnino, C. R. Simões, I. M. D. Torres, D. Varela, J. P. Zurano, P. A. M. Marques, and L. Dos Anjos. 2024. Acoustic monitoring of anurans and birds in tropical biomes. Biotropica 56(3):e13307. https://doi.org/10.1111/btp.13307
De Camargo, U., T. Roslin, and O. Ovaskainen. 2019. Spatio-temporal scaling of biodiversity in acoustic tropical bird communities. Ecography 42:1936-1947. https://doi.org/10.1111/ecog.04544
Dooling, R. J. 2004. Audition: can birds hear everything they sing? Pages 206-224 in P. Marler and H. Slabbekoorn, editors. Nature’s music, the science of birdsong. Elsevier Academic Press, San Diego, California, USA. https://doi.org/10.1016/B978-012473070-0/50010-4
Dos Anjos, L., H. S. Oliveira, G. Willrich, M. Jardim, G. L. M. Rosa, and C. B. de Araújo. 2022, Atlantic Forest birds are more functionally and taxonomically diverse in valley bottoms relative to uplands. Acta Oecologica 115:103829 https://doi.org/10.1016/j.actao.2022.103829
Hart, P. J., R. Hall, W. Ray, A. Beck, and J. Zook J. 2015. Cicadas impact bird communication in a noisy tropical rainforest. Behavioral Ecology 26(3):839-842. https://doi.org/10.1093/beheco/arv018
Henwood, K., and A. Fabrick. 1979. A quantitative analysis of the dawn chorus: temporal selection for community optimization. American Naturalist 114(2):260-274. https://doi.org/10.1086/283473
Hopping, W. A., C. J. Sayers II, N. R. Huaraca-Charca, and H. Klinck. 2024. Simultaneous passive acoustic monitoring uncovers evidence of potentially overlooked temporal variation in an Amazonian bird community. Ibis 166(3):986-1002. https://doi.org/10.1111/ibi.13293
Hutschenreiter, A., E. Andresen, M. Briseño-Jaramillo, A. Torres-Araneda, E. Pinel-Ramos, J. Baier, and F. Aureli. 2024. How to count bird calls? Vocal activity indices may provide different insights into bird abundance and behaviour depending on species traits. Methods in Ecology and Evolution 15(6):1071-1083. https://doi.org/10.1111/2041-210X.14333
Jorge, F. C., C. G. Machado, S. S. da Cunha Nogueira, and S. L. G. Nogueira-Filha. 2018. The effectiveness of acoustic indices for forest monitoring in Atlantic rainforest fragments. Ecological Indicators 91:71-76. https://doi.org/10.1016/j.ecolind.2018.04.001
Karr, J. R., S. K. Robinson, J. G. Blake, and R. O. Bierregaard, Jr. 1990. Birds of four Neotropical forests. Pages 237-269 in A. Gentry, editor. Four Neotropical rainforests. Yale University Press, New Haven, Connecticut, USA.
Leach, E. C., C. J. Burwell, L. A. Ashton, D. N. Jones, and R. L. Kitching. 2016. Comparison of point counts and automated acoustic monitoring: detecting birds in a rainforest biodiversity survey. Emu 116(3):305-309. https://doi.org/10.1071/MU15097
Luther, D. 2008. Signaller:receiver coordination and the timing of communication in Amazonian birds. Biology Letters 4(6):651-654. https://doi.org/10.1098/rsbl.2008.0406
Luther, D. 2009. The influence of the acoustic community on songs of birds in a neotropical rain forest. Behavioral Ecology 20(4):864-871. https://doi.org/10.1093/beheco/arp074
Menger, J., W. E. Magnusson, M. J. Anderson, M. Schlegel, G. Pe'er, and K. Henle. 2017. Environmental characteristics drive variation in Amazonian understorey bird assemblages. PLoS ONE 12:e0171540 https://doi.org/10.1371/journal.pone.0171540
Metcalf, O. C., J. Barlow, S. Marsden, N. G. de Moura, E. Berenguer, J. Ferreira, and A. C. Lees. 2022. Optimizing tropical forest bird surveys using passive acoustic monitoring and high temporal resolution sampling. Remote Sensing in Ecology and Conservation 8(1):45-56. https://doi.org/10.1002/rse2.227
Oliveira, H. S., I. A. Barreto, and L. dos Anjos. 2023. Temporal beta diversity of bird species using the point count method indicates predominance of turnover over nestedness in an Atlantic Forest site. Ornithology Research 31:265-273. https://doi.org/10.1007/s43388-023-00147-z
Parker III, T. E. 1991. On the use of tape recorders in avifaunal surveys. Auk 108:443-444.
Pérez-Granados, C., and J. Traba. 2021. Estimating bird density using passive acoustic monitoring: a review of methods and suggestions for further research. Ibis 163(3):765-783. https://doi.org/10.1111/ibi.12944
Pollock, H. S., J. D. Toms, C. E. Tarwater, T. J. Benson, J. R. Karr, and J. D. Brawn. 2022. Long-term monitoring reveals widespread and severe declines of understory birds in a protected Neotropical forest. Proceedings National Academy Sciences 119(16):e2108731119. https://doi.org/10.1073/pnas.2108731119
Pomara, L. Y., K. Ruokolainen, H. Tuomisto, and K. R. Young. 2012. Avian composition co-varies with floristic composition and soil nutrient concentration in Amazonian upland forests. Biotropica 44(4):545-553. https://doi.org/10.1111/j.1744-7429.2011.00851.x
Ribeiro Jr., J. W., L. S. M. Sugai, and M. Campos-Cerqueira. 2017. Passive acoustic monitoring as a complementary strategy to assess biodiversity in the Brazilian Amazonia. Biodiversity Conservation 26:299-302. https://doi.org/10.1007/s10531-017-1390-0
Robinson, W. D., J. D. Brawn, and S. K. Robinson. 2000. Forest bird community structure in central Panama: influence of spatial scale and biogeography. Ecological Monographs 70(2):209-235. https://doi.org/10.1890/0012-9615(2000)070[0209:FBCSIC]2.0.CO;2
Ross, S. R. P.-J., D. P. O’Connell, J. L. Deichmann, C. Desjonquères, A. Gasc, J. N. Phillips, S. S. Sethi, C. M. Wood, and Z. Burivalova. 2023. Passive acoustic monitoring provides a fresh perspective on fundamental ecological questions. Functional Ecology 37(4):959-975. https://doi.org/10.1111/1365-2435.14275
Sebastián-González, E., R. J. Camp, A. M. Tanimoto, P. M. de Oliveira, B. B. Lima, T. A. Marques, and P. J. Hart. 2018. Density estimation of sound-producing terrestrial animals using single automatic acoustic recorders and distance sampling. Avian Conservation and Ecology 13(2):7https://doi.org/10.5751/ACE-01224-130207
Skutch, A. F. 1954. Life histories of Central American birds. Families Fringillidae, Thraupidae, Icteridae, Parulidae and Coerebidae. Pacific Coast Avifauna No. 31. https://doi.org/10.5962/bhl.title.155273
Skutch, A. F. 1960. Life histories of Central American birds II. Families Vireonidae, Sylviidae, Turdidae, Troglodytidae, Paridae, Corvidae, Hirundinidae and Tyrannidae. Pacific Coast Avifauna No. 34.
Stevens, H. C., E. M. Metz, P. S. Del Castillo, J. D. Alván, and M. T. Bowler. 2019. Use of autonomous audio recordings for the rapid inventory of birds in the white-sand forests of the Peruvian Amazon. Journal of Field Ornithology 90(1):70-79. https://doi.org/10.1111/jofo.12279
Stouffer, P. C., V. Jirinec, C. L. Rutt, R. O Bierregaard Jr., A. Hernández-Palma, E. I. Johnson, S. R. Midway, L. L. Powell, J. D. Wolfe, and T. E. Lovejoy. 2020. Long-term change in the avifauna of undisturbed Amazonian rainforest: ground-foraging birds disappear and the baseline shifts. Ecology Letters 24(2):186-195. https://doi.org/10.1111/ele.13628
Sugai, L. S. M., T. S. F. Silva, J. W. Ribeiro Jr., and D. Llusia. 2019. Terrestrial passive acoustic monitoring: review and perspectives. BioScience 69(1):15-25. https://doi.org/10.1093/biosci/biy147
Terborgh, J., S. K. Robinson, T. A. Parker III, C. A. Munn, and N. Pierpont. 1990. Structure and organization of an Amazonian forest bird community. Ecological Monographs 60(2):213-238. https://doi.org/10.2307/1943045
Tobias, J. A., R. Planqué, D. L. Cram, and N. Seddon. 2014. Species interactions and the structure of complex communication networks. Proceeding National Academy Sciences 111(3):1020-1025. https://doi.org/10.1073/pnas.1314337111
Weir, J. T., D. J. Wheatcroft, and T. D. Price. 2012. The role of ecological constraint in driving the evolution of avian song frequency across a latitudinal gradient. Evolution 66(9):2773-2783 https://doi.org/10.1111/j.1558-5646.2012.01635.x
Winiarska, D., P. Szymański, and T. S. Osiejuk. 2024. Detection ranges of forest bird vocalisations: guidelines for passive acoustic monitoring. Scientific Reports 14:894. https://doi.org/10.1038/s41598-024-51297-z
Fig. 1
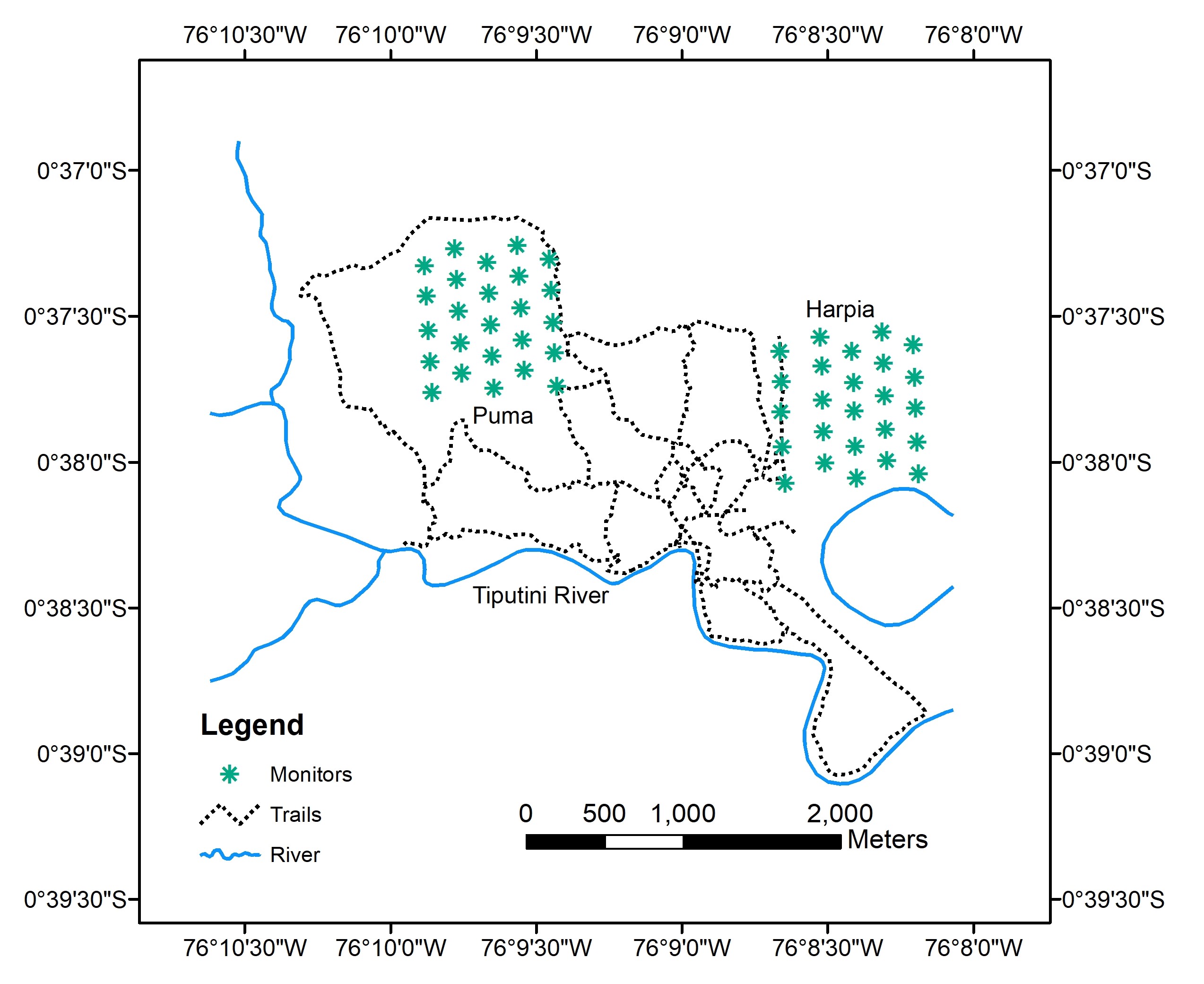
Fig. 1. Map of Tiputini Biodiversity Station, Ecuador, showing locations of ARUs on two 100 ha study plots (Puma, Harpia). This map was originally published in Blake (2021).
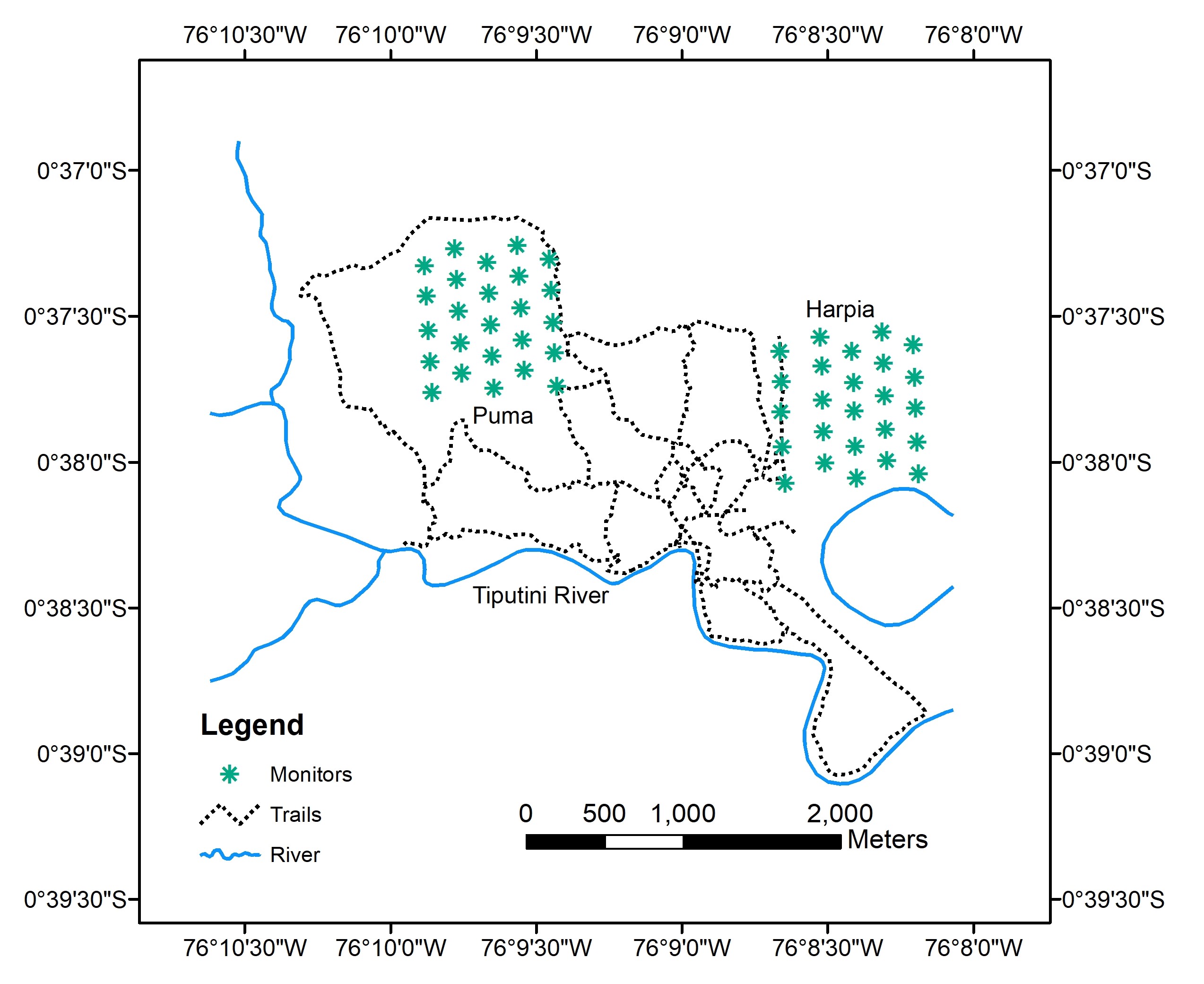
Fig. 2
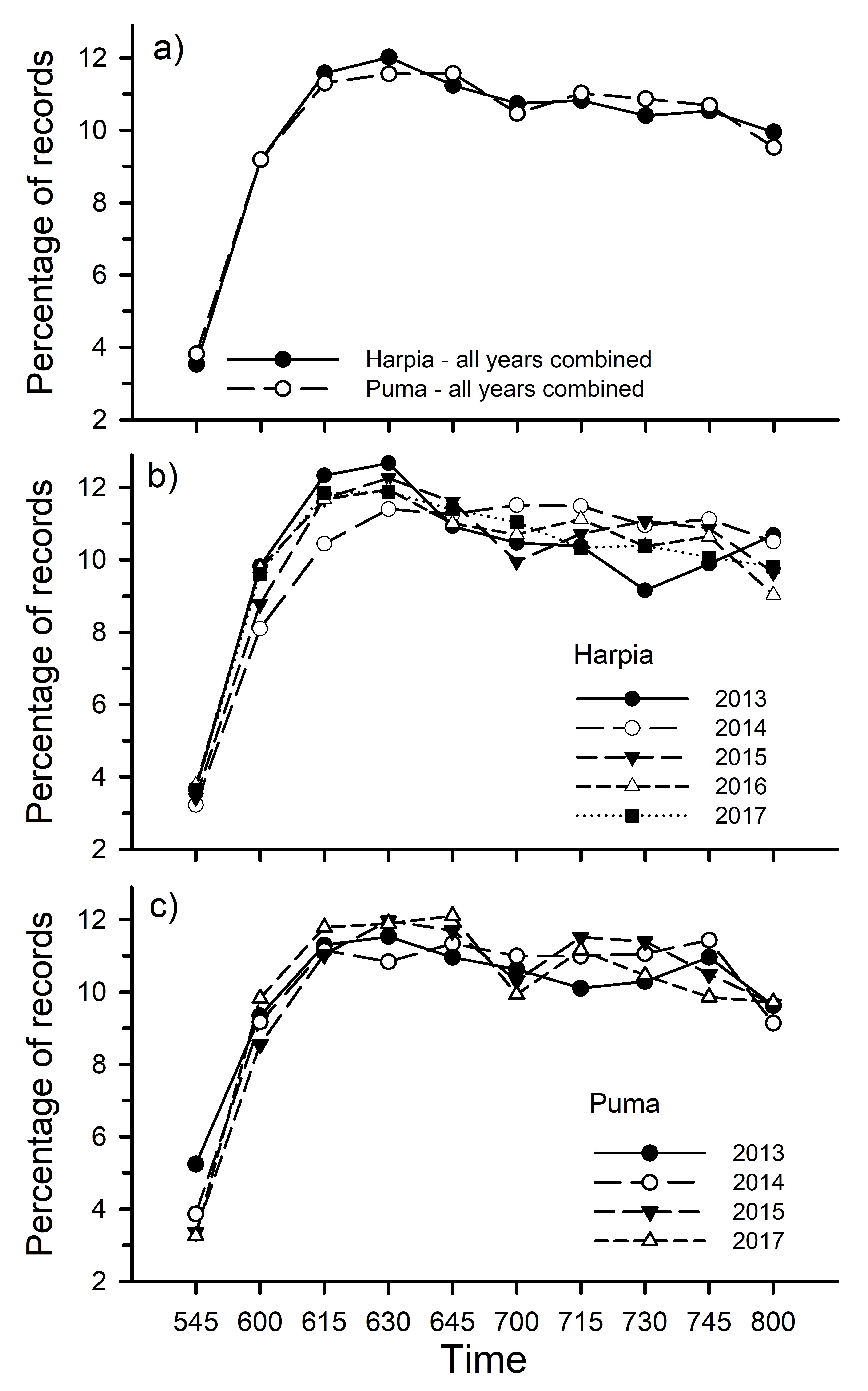
Fig. 2. Percentage of vocal activity records by time (10-min periods) based on all records, including unidentified species, for both plots with all years combined (a) and for Harpia (b) and Puma (c) by year.
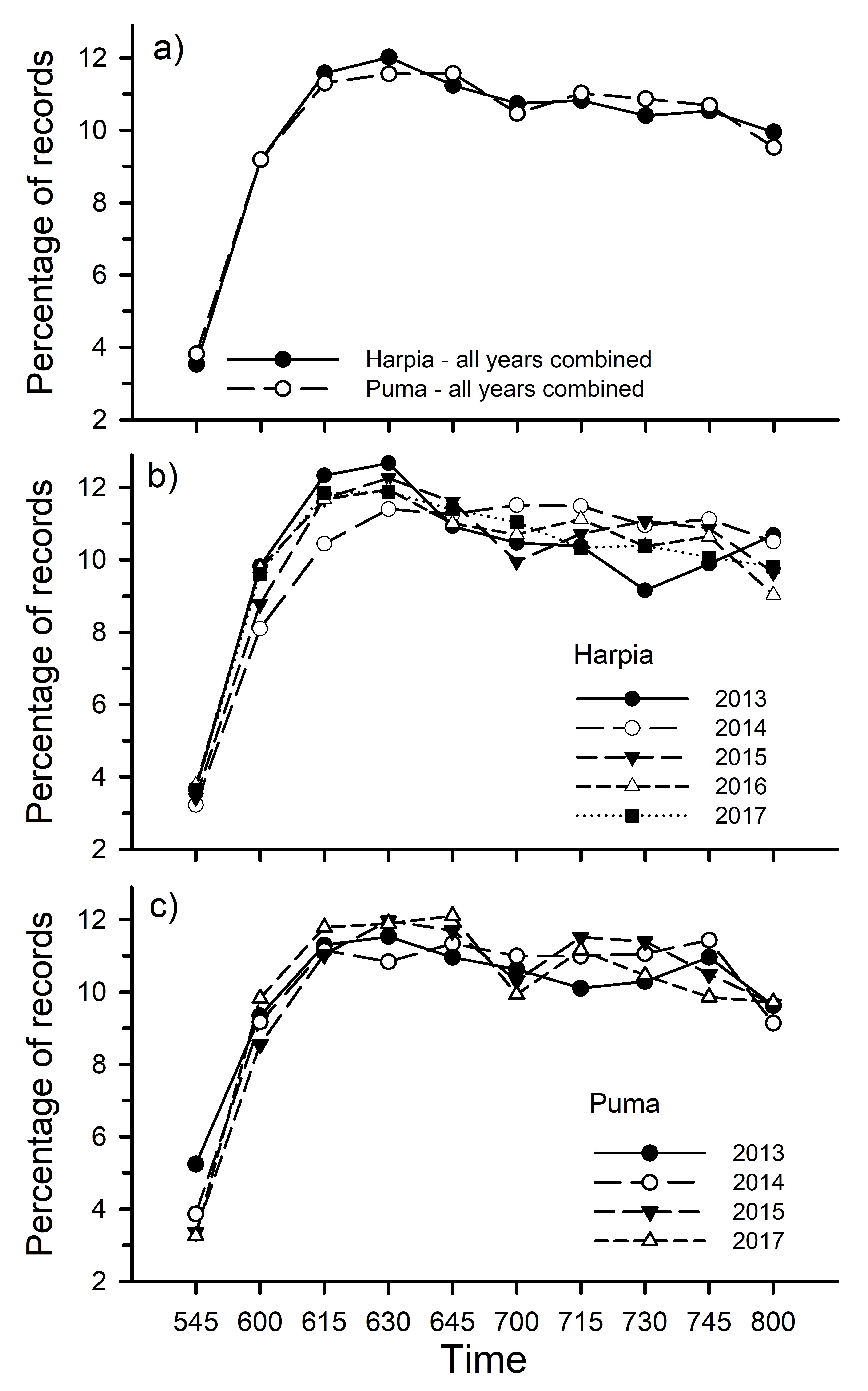
Fig. 3
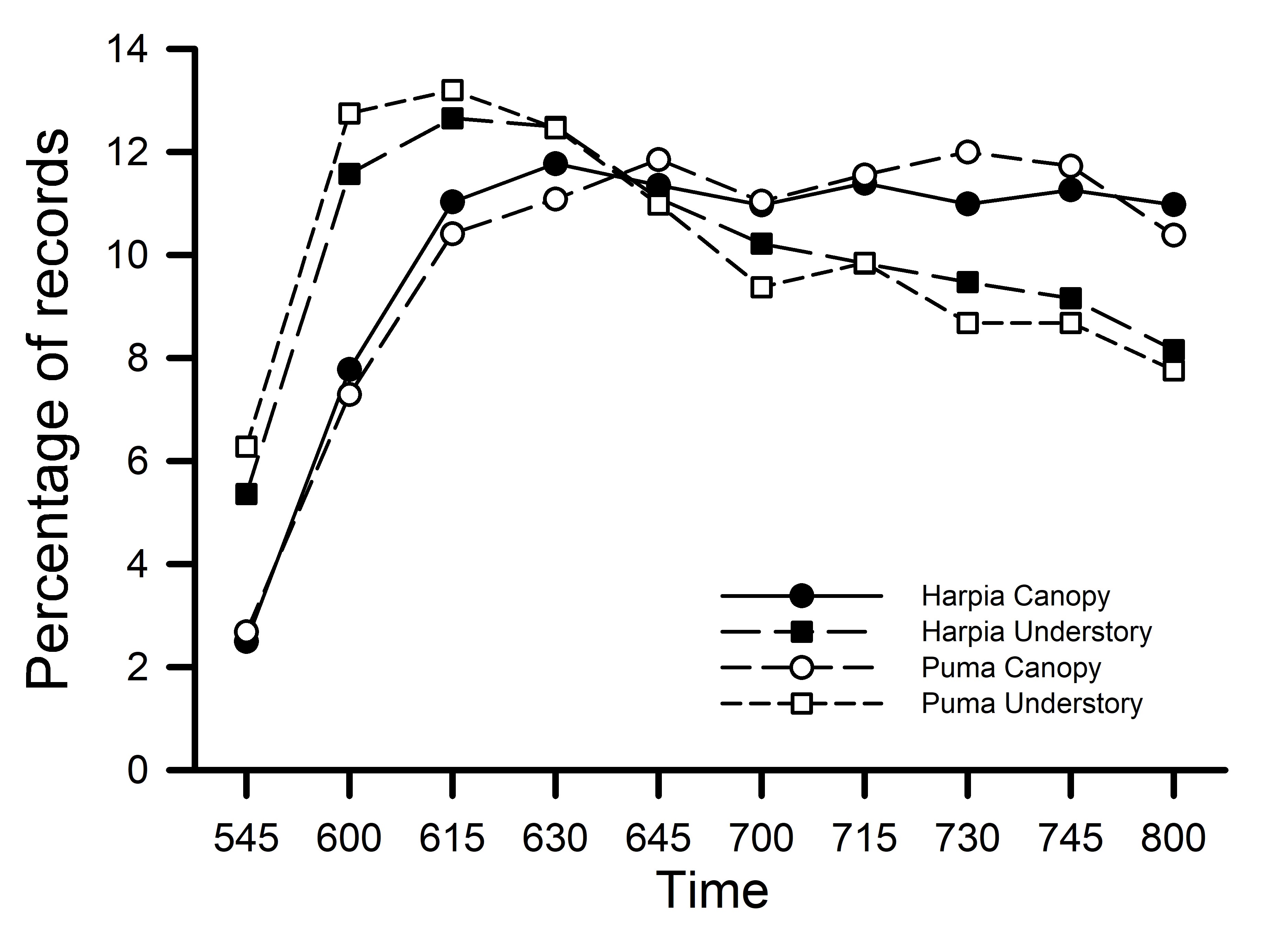
Fig. 3. Percentage of vocal activity records by time (10-min periods) on each plot for canopy and understory species. Foraging strata (canopy, including subcanopy; understory, including ground) follows Karr et al. (1990).
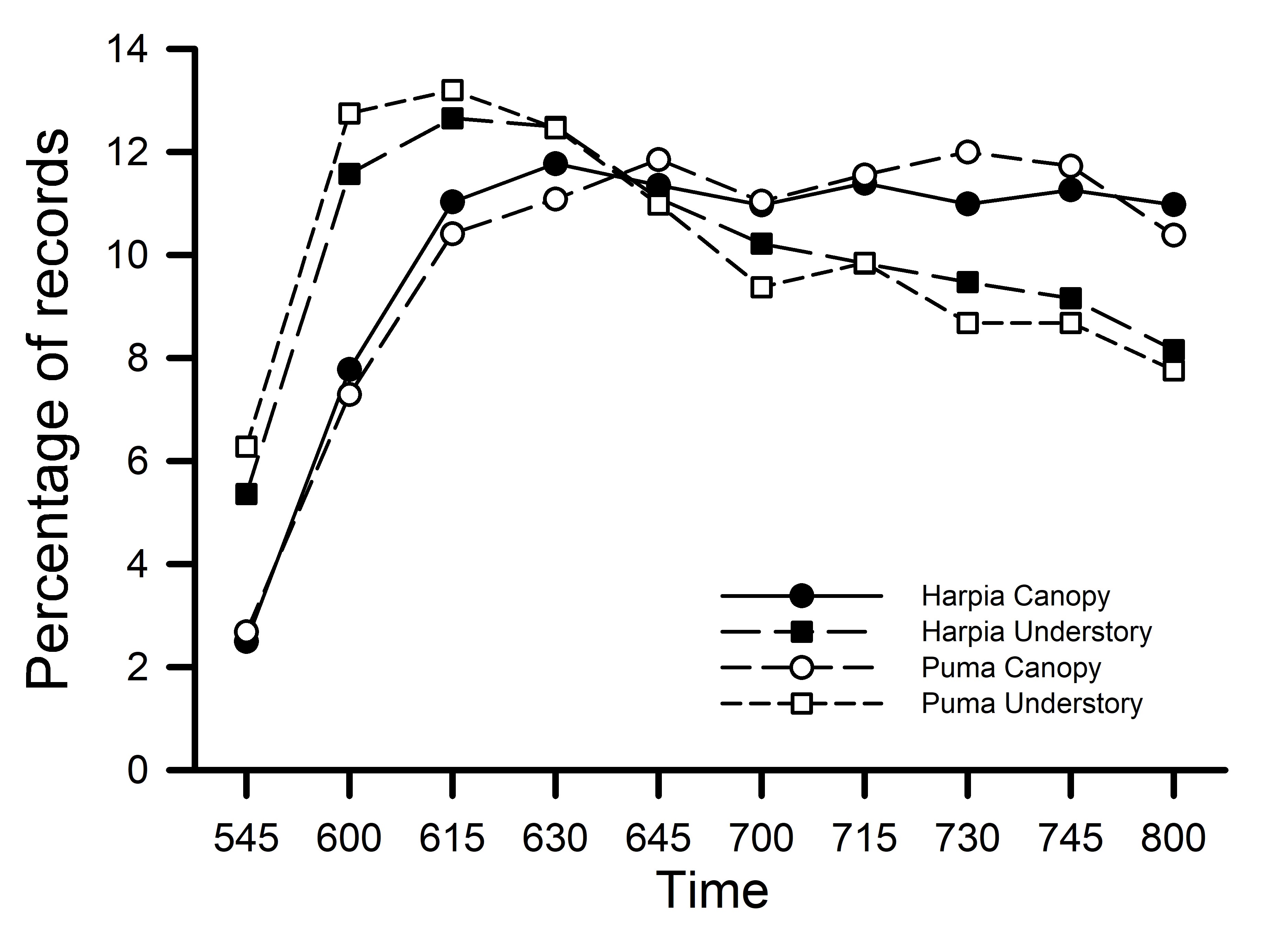
Fig. 4
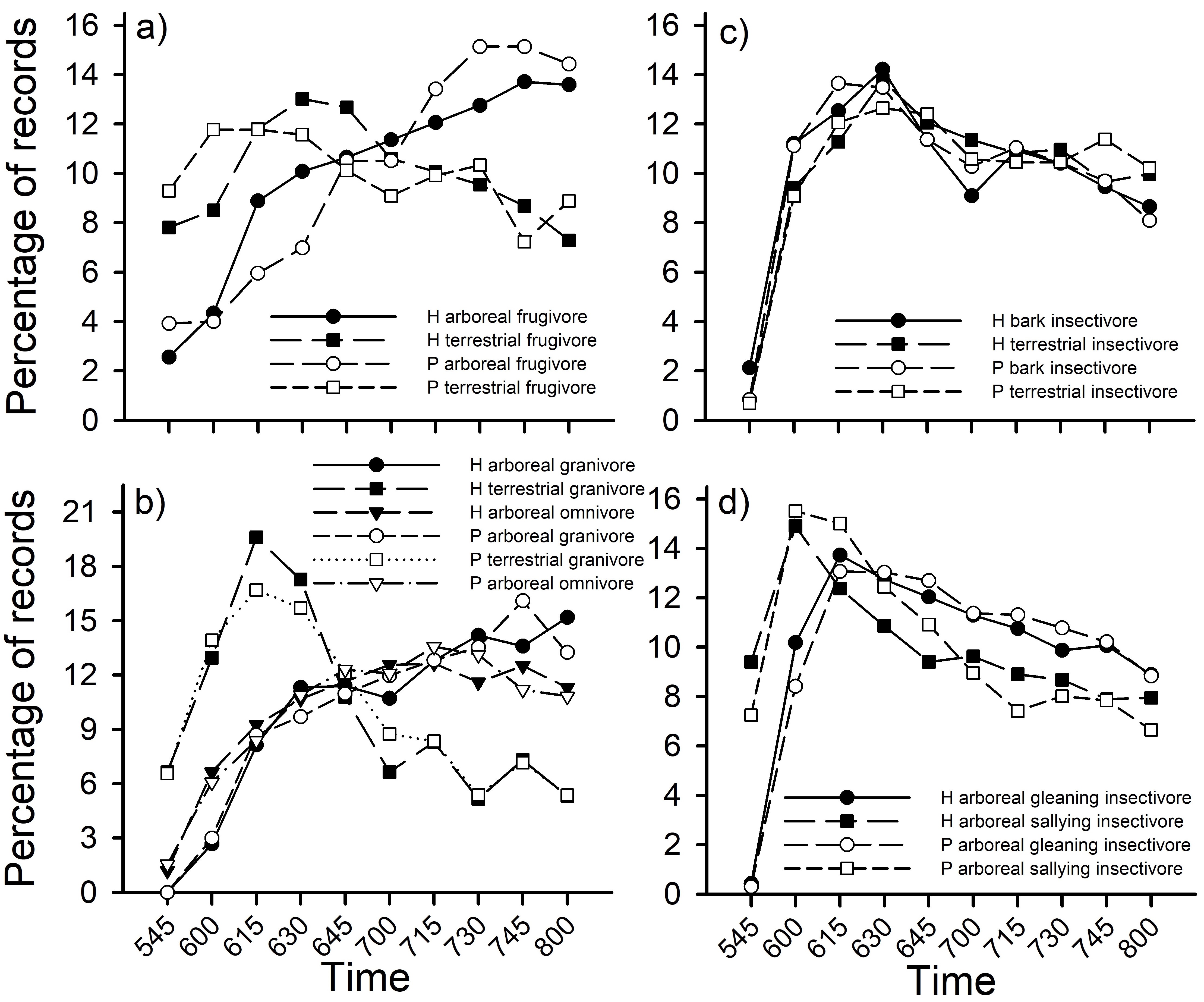
Fig. 4. Percentage of vocal activity records by time (10-min periods) on each plot (Harpia – H; Puma -P) for different sets of foraging guilds: a) frugivores, b) bark and terrestrial insectivores, c) granivores and omnivores, and d) gleaning and sallying insectivores. Guild designations follow Terborgh et al. (1990).
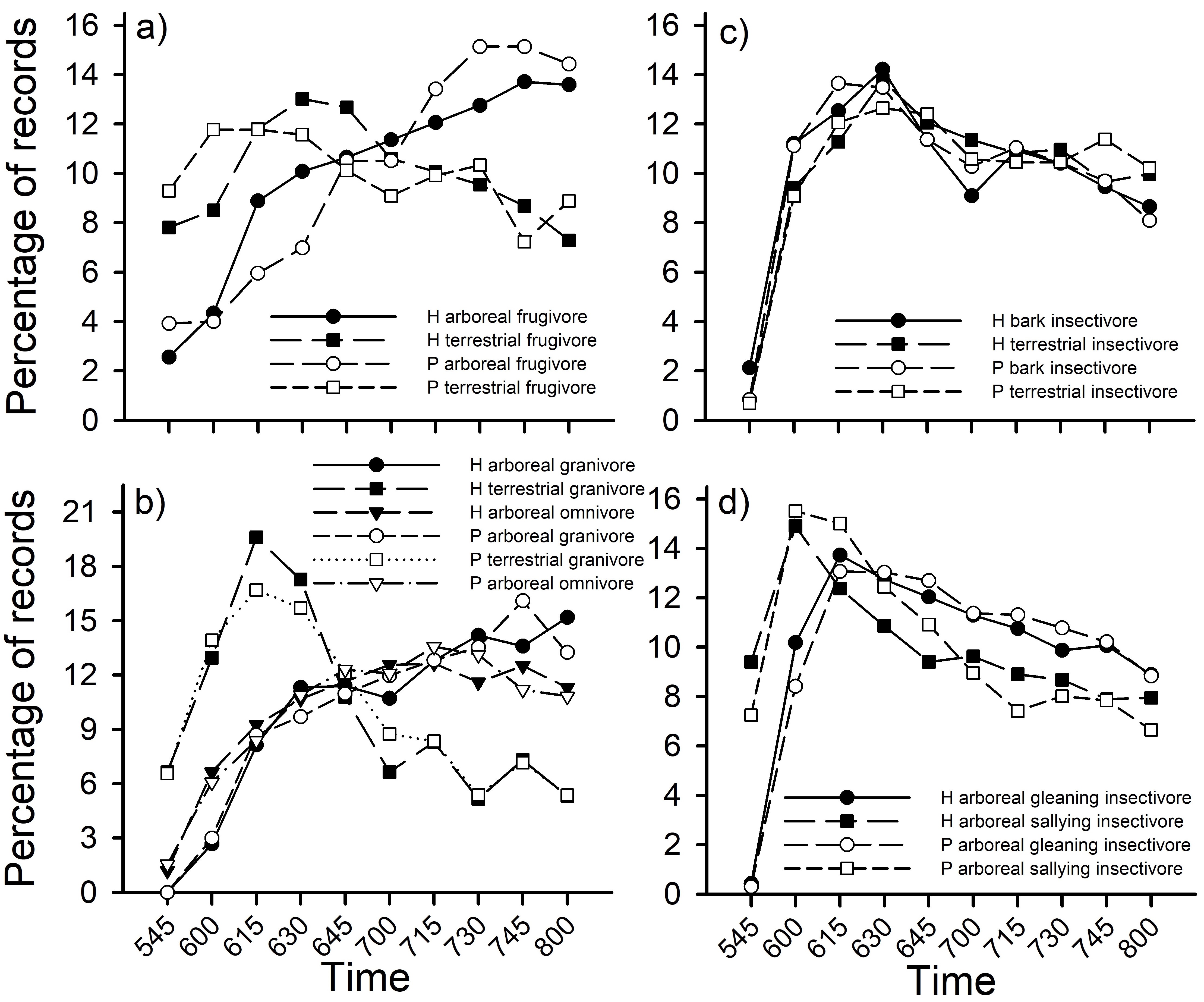
Fig. 5
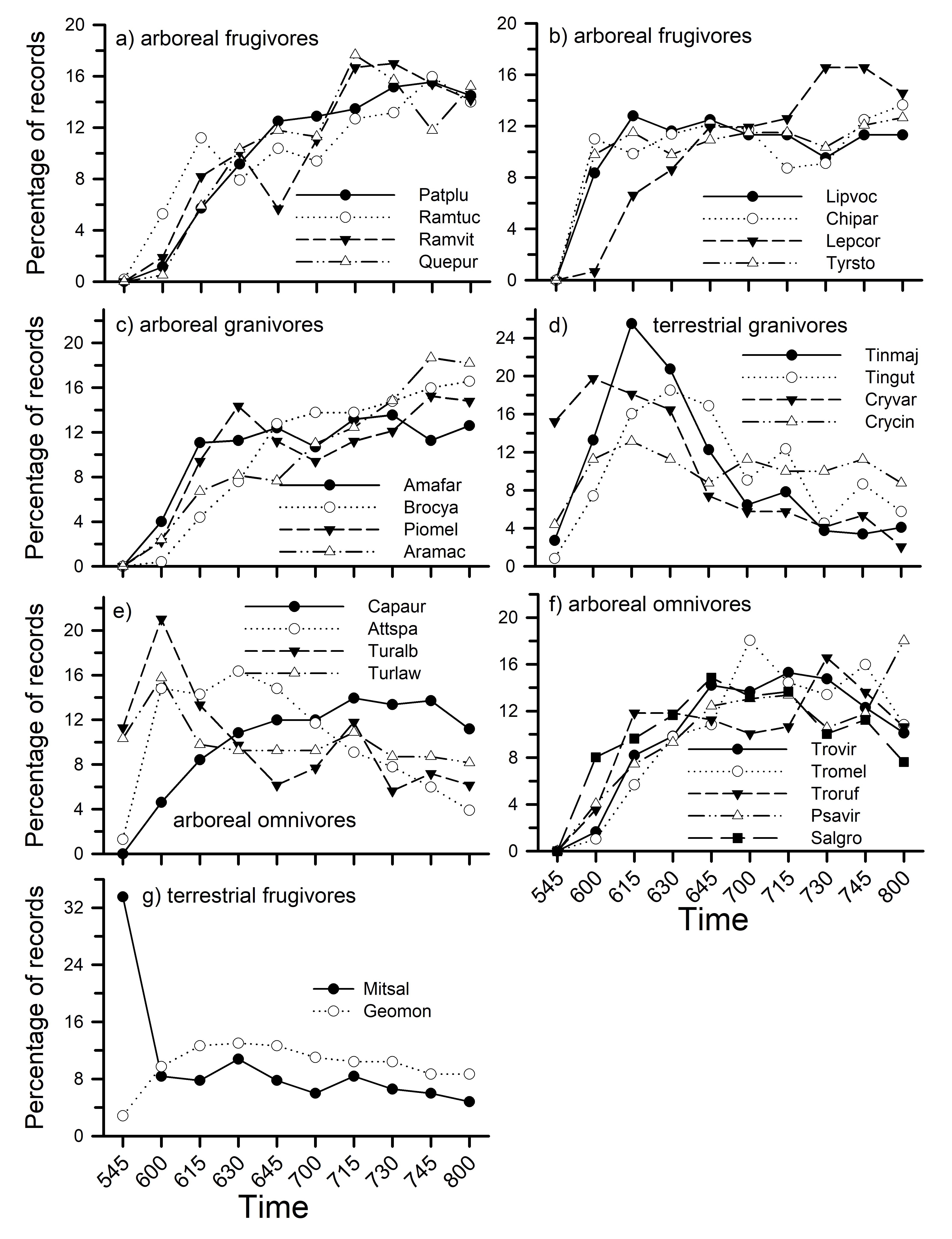
Fig. 5. Percentage of vocal activity records by time (10-min periods) on each plot for different frugivores, granivores, and omnivores, following Terborgh et al. (1990). Species codes: Amafar – Amazona farinosa, Aramac – Ara macao, Attspa – Attila spadiceus, Brocya – Brotogeris cyanoptera, Capaur – Capito auratus, Chipar – Chiroxiphia pareola, Crycin – Crypturellus cinereus, Cryvar – C. variegatus, Geomon – Geotrygon montana, Lepcor – Lepidothrix coronata, Lipvoc – Lipaugus vociferans, Mitsal – Mitu salvini, Patplu - Patagioenas plumbea, Piomel – Pionites melanocephalus, Psavir – Psaracolius viridis, Quepur – Querula purpurata, Ramtuc – Ramphastos tucanus, Ramvit – R. vitellinus, Salgro – Saltator grossus, Tingut – Tinamus guttatus, Tinmaj – T. major, Tromel – Trogon melanurus, Troruf – T. rufus, Trovir – T. viridis, Turalb – Turdus albicollis, Turlaw – T. lawrencii, Tyrsto – Tyranneutes stolzmanni.
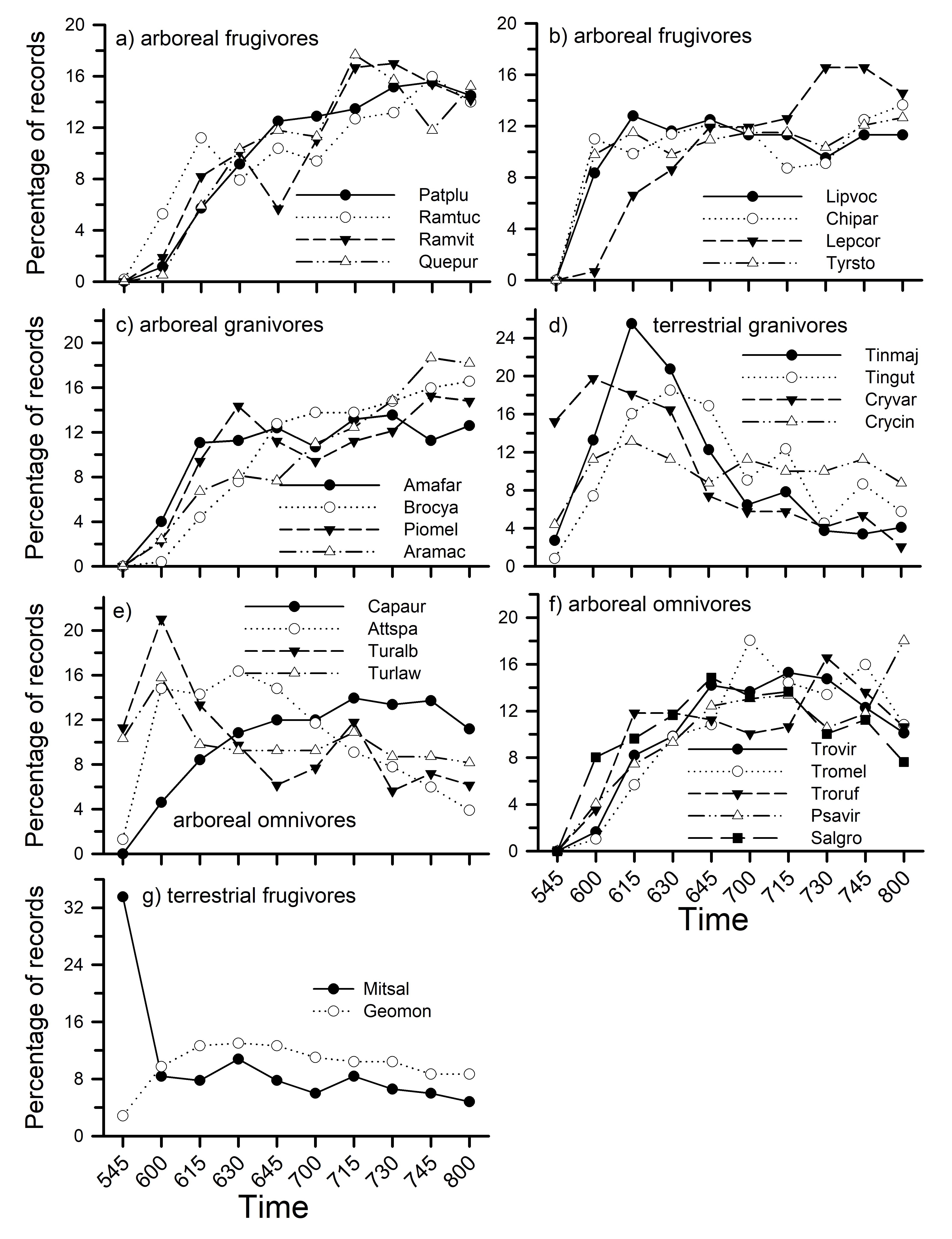
Fig. 6
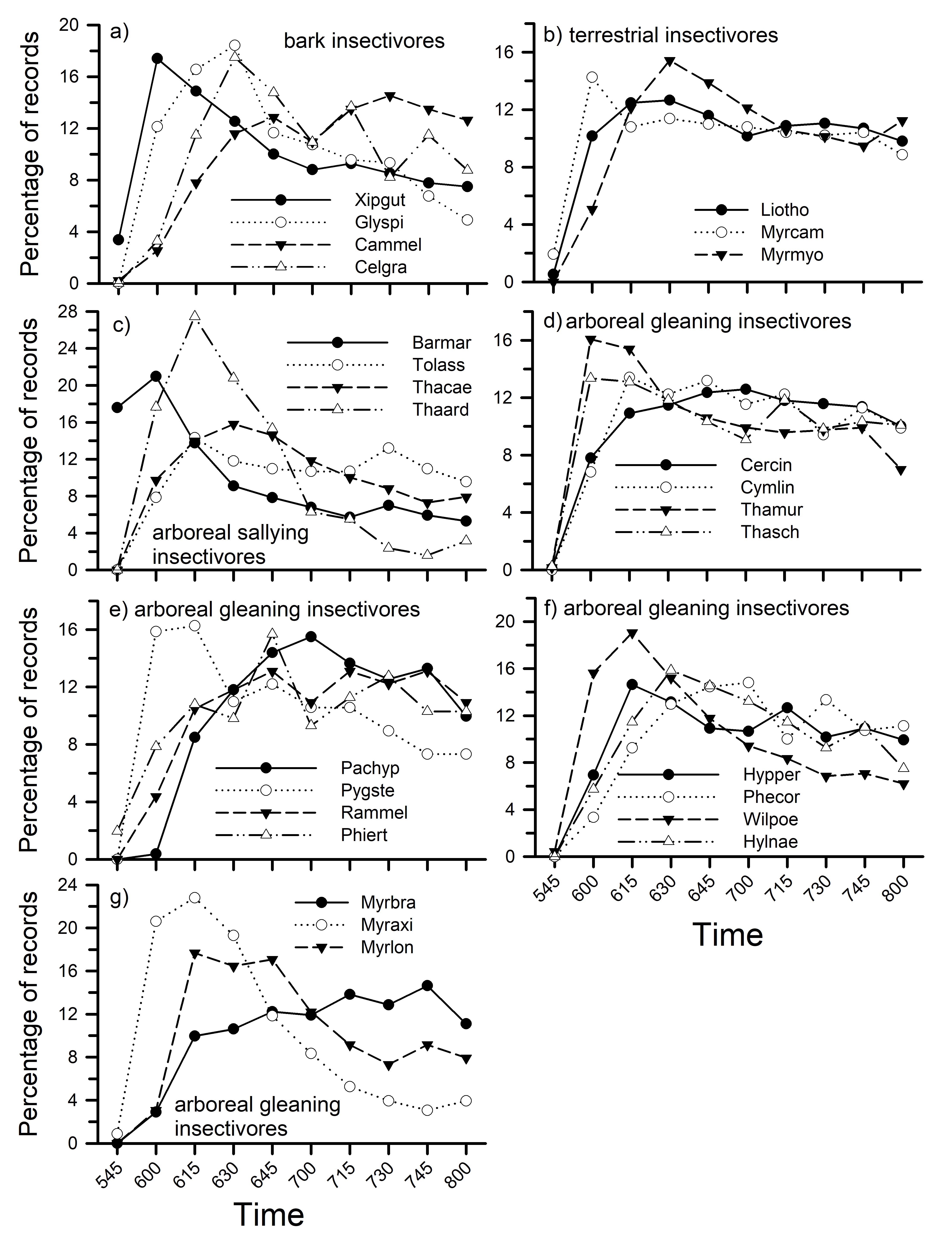
Fig. 6. Percentage of vocal activity records by time (10-min periods) on each plot for different insectivores, following Terborgh et al. (1990). Species codes: Barmar - Baryphthengus martii, Cammel – Campephilus melanoleucos, Celgra – Celeus grammicus, Cercin – Cercomacra cinerescens, Cymlin – Cymbilaimus lineatus, Glyspi – Glyphorynchus spirurus, Pachyp – Pachysylvia hypoxantha, Hylnae – Hylophylax naevia, Hypper – Hypocnemis peruviana, Liotho – Liosceles thoracicus, Myraxi – Myrmotherula axillaris, Myrbra – M. brachyura, Myrcam – Myrmothera campanisona, Myrlon – Myrmotherula longipennis, Myrmyo – Myrmoborus myotherinus, Phecor – Pheugopedius coraya, Phiert – Philydor erythroptera, Pygste – Pygiptila stellaris, Rammel – Ramphocaenus melanurus, Thaard – Thamnomanes ardesiacus, Thacae – T. caesius, Thamur – Thamnophilus murinus, Thasch – T. schistaceus, Tolass – Tolmomyias assimilis, Wilpoe – Willisornis poecilinotus, Xipgut – Xiphorhychus guttatus.
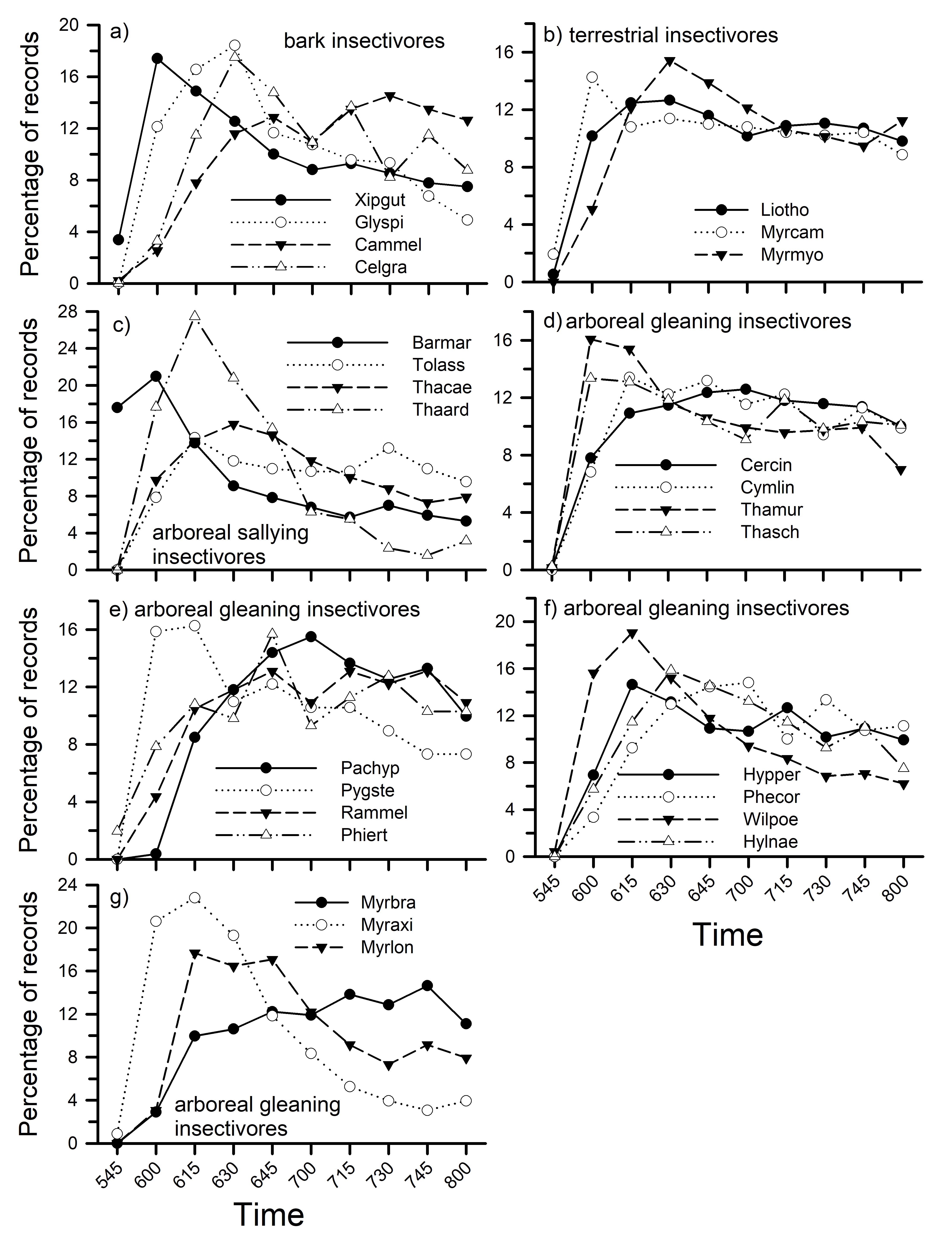
Table 1
Table 1. Numbers of species with different ranges of records of activity. Data were combined from two 100-ha study plots (Harpia, Puma) at Tiputini Biodiversity Station, Ecuador.
Range of records | Species | % of total species | Records | % of total records | |||||
>499 | 12 | 5.5 | 8658 | 32.7 | |||||
400-499 | 7 | 3.2 | 3125 | 11.8 | |||||
300-399 | 8 | 3.7 | 2809 | 10.6 | |||||
200-299 | 16 | 7.4 | 3860 | 14.6 | |||||
150-199 | 14 | 6.5 | 2434 | 9.2 | |||||
100-149 | 12 | 5.5 | 1462 | 5.5 | |||||
50-99 | 34 | 15.7 | 2499 | 9.4 | |||||
1-49 | 114 | 52.5 | 1667 | 6.3 | |||||
1-10 | 62 | 28.6 | 269 | 1.0 | |||||